The UNH CME group has many years of experience working on CME impacts on planetary magnetospheres, specifically on Mercury’s and Earth’s magnetosphere.
Impacts on Mercury’s magnetosphere
Mercury is the only planet in the inner solar system, other than Earth, that possesses a dynamo-generated global, albeit weak, magnetic field. Mercury’s proximity to the Sun, with a heliocentric distance between 0.31 and 0.47 au, results in more extreme solar wind and interplanetary conditions than at any other planet in our solar system. The interaction between this weak planetary magnetic field and the strong solar wind conditions creates a highly dynamic magnetosphere that is pushed to its extremes during CME passages. On average, Mercury’s magnetopause is 15% closer to the planet during CME conditions than under nominal solar wind (Winslow et al., 2017), while during more extreme CME events, the dayside magnetosphere completely disappears (Figure 7 below) as the magnetosphere is compressed below the surface of the planet (Winslow et al., 2020). Such a collapse of Mercury’s dayside magnetosphere leads to CME plasma reaching Mercury’s surface directly. Furthermore, the collapse of Mercury’s dayside magnetosphere has important implications for the habitability of close-in exoplanets around M dwarf stars, as such events may significantly contribute to planetary atmospheric loss in these systems.
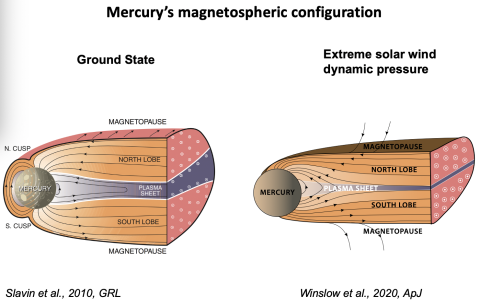
Figure 7. Cartoon depictions of Mercury’s magnetosphere under nominal solar wind conditions (left) and under extreme solar wind conditions caused by a CME (right).
Impacts on Earth’s magnetosphere
The multi-faceted response of the terrestrial magnetosphere/magnetosheath to CME passage has been the subject of research for about 4 decades. Here, we can only touch on a few aspects of this voluminous work. From a statistical perspective (see e.g. Farrugia et al., 2006), the most perturbed state of the magnetosphere is during the maximum phase of the solar cycle where CMEs dominate.
During their long (~1-2 day) passage at Earth, the interplanetary fields of CMEs and magnertic cloud change slowly and reach extreme values hardly otherwise ever sampled (e.g., see Farrugia et al., 1993a). We can thus monitor the evolution of Earht’s magnetosphere as it experiences a wide range of stable parameters. Among these interplanetary extreme states are (i) large, positive Bz, (ii) large negative Bz, (iii) a magnetic field dominated by its east-west component, By, and (iv) low plasma beta and low Alfven Mach number. The long duration of ICME/MC passage can imply (depending on the orientation) long intervals of negative Bz, and this gives rise to repetitive, sawtooth substorms (see Farrugia et al., 1993b).
During MC passage, values of the upstream Alfven Mach number are much lower than those otherwise sampled (~3 versus 8-10) as discussed in Farrugia et al. (1995) and Lavraud and Borovsky (2008), among others. Together with the magnetic shear across the magnetopause, this parameter plays a central role in determining the structure of the magnetosheath close to the magnetopause. In addition, when the upstream Alfven Mach number is low, the existence of a plasma depletion layer is predicted, irrespective of the magnetic shear across the magnetopause. In extreme case (Lugaz et al., 2015b), the solar wind Mach number may become sub-Alfvénic, which results in the disappearance of Earth’s bow shock.
Fast CMEs drive shocks. The shock is itself a good agent for enhancing preceding Bz < 0 solar wind fields. Thus, CME arrival at Earth would be expected to elicit a two-step response (Kamide et al., 1998): the storm time Dst index would drop during the passage of the sheath, recover for a few hours, and then drop again. This is the classic shock - driver cause of double-dip strong storms. Geoeffects of shocks seen in association with CMEs (not only those driven by CMEs but also those propagating inside a CME) have been the subject of recent attention (see Lugaz et al., 2015a, 2016; Kilpua et al., 2017).
Analyzing an extreme event associated with the interaction of two CMEs, Farrugia et al (2006b) argued that the magnetosphere ‘‘senses’’ the presence of the two ejecta and responds with a reactivation of the ring current soon after it started to recover from the passage of the first ejection. A drift-loss global kinetic model of ring current buildup showed that in this case the major factor determining the intensity of the storm activity was the very high (up to ~10 cm-3) plasma sheet density. The plasma sheet density, in turn, was found to correlate well with the very high solar wind density, suggesting the compression of the leading ejecta as the source of the hot, superdense plasma sheet in this case. In this example, there resulted a double-dip great storm (each minimum Dst < -250 nT) not due to a typical sheath and ejecta field but to two interacting ejecta.